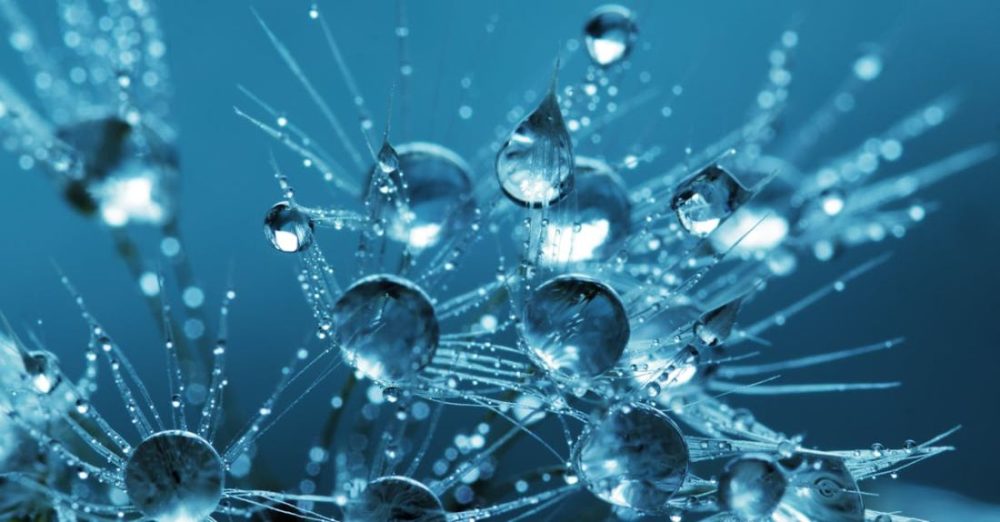
Computational modeling has revolutionized the field of cell analysis, providing researchers with powerful tools to explore complex biological systems in ways that were previously impossible. By merging mathematical algorithms and computer simulations, computational modeling offers a detailed and dynamic perspective on cellular processes, shedding light on intricate mechanisms that govern cell behavior. This article delves into the applications of computational modeling in cell analysis, showcasing its significance in advancing our understanding of fundamental cellular functions.
**Understanding Cellular Dynamics**
One of the primary applications of computational modeling in cell analysis is to unravel the intricate dynamics of cellular processes. Cells are dynamic entities that constantly interact with their environment, responding to external stimuli and undergoing complex signaling pathways. Computational models enable researchers to simulate these dynamic interactions, providing insights into how cells communicate, proliferate, differentiate, and respond to various stimuli at the molecular level. By integrating experimental data with computational simulations, scientists can gain a comprehensive understanding of the spatiotemporal dynamics within cells, unraveling the complexities of cellular behavior.
**Predicting Cellular Responses**
Computational modeling plays a crucial role in predicting cellular responses to different stimuli, drugs, or genetic perturbations. By developing predictive models based on known biological principles, researchers can simulate how cells would react under various conditions, helping to identify potential targets for therapeutic interventions or understand the mechanisms underlying diseases. These predictive models can also aid in optimizing drug dosages, predicting drug resistance, or designing personalized treatment strategies tailored to individual patients based on their cellular characteristics. By harnessing the power of computational modeling, researchers can accelerate the discovery of novel therapeutic approaches and enhance precision medicine initiatives.
**Exploring Signaling Pathways**
Cellular signaling pathways regulate a myriad of biological processes, including cell growth, differentiation, and apoptosis. Computational modeling allows researchers to dissect these intricate signaling networks, predicting how different components interact and influence cellular outcomes. By constructing mathematical models that capture the dynamics of signaling pathways, scientists can unravel the crosstalk between various signaling molecules, identify key regulators, and predict the consequences of perturbations within the network. This computational approach not only enhances our understanding of signaling dynamics but also provides a platform for exploring potential therapeutic targets for diseases associated with dysregulated signaling pathways.
**Simulating Cell Migration and Morphogenesis**
Cell migration and morphogenesis are fundamental processes essential for embryonic development, tissue regeneration, and immune responses. Computational modeling offers a valuable tool for simulating and analyzing the complex behaviors associated with cell migration and morphogenesis. By integrating biophysical principles with computational algorithms, researchers can model how cells move, adhere, and deform in response to mechanical cues or chemical gradients. These simulations provide insights into the underlying mechanisms driving cell migration, tissue patterning, and organ development, shedding light on the fundamental principles governing morphogenetic processes.
**Optimizing Bioprocesses**
In addition to its applications in fundamental cell biology, computational modeling is also instrumental in optimizing bioprocesses for various applications, such as biopharmaceutical production, tissue engineering, and regenerative medicine. By developing computational models that simulate cell growth, metabolism, and product formation, researchers can optimize bioreactor designs, culture conditions, and process parameters to enhance cell productivity and product quality. Computational modeling allows for the rapid screening of different scenarios, guiding experimental efforts and accelerating the development of bioprocesses with improved efficiency and scalability.
**Unlocking the Potential of Single-Cell Analysis**
Recent advancements in single-cell analysis have provided unprecedented insights into cellular heterogeneity and diversity. Computational modeling complements single-cell experimental techniques by enabling the integration and interpretation of large-scale single-cell datasets. By developing computational frameworks that analyze single-cell omics data, researchers can identify cellular subpopulations, infer regulatory networks, and unravel the dynamics of gene expression at the single-cell level. These integrative approaches enhance our understanding of cellular heterogeneity and provide a holistic view of complex biological systems, paving the way for new discoveries in cell biology and personalized medicine.
**In Summary**
Computational modeling has emerged as a powerful tool for advancing cell analysis, offering unique insights into the dynamics, behavior, and interactions of cells at multiple scales. By combining mathematical modeling with experimental data, researchers can simulate complex cellular processes, predict cellular responses, explore signaling pathways, simulate morphogenetic events, optimize bioprocesses, and unlock the potential of single-cell analysis. The integration of computational modeling in cell analysis not only enhances our understanding of fundamental biological processes but also accelerates the development of innovative therapeutic strategies and personalized interventions. As computational modeling continues to evolve, its impact on cell analysis is poised to drive transformative discoveries and shape the future of biomedical research.